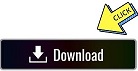
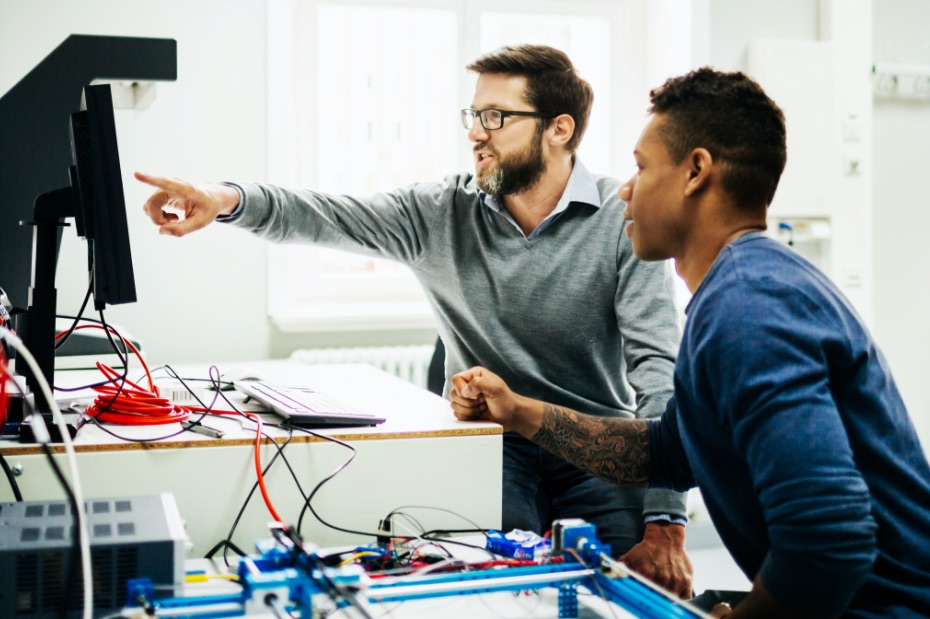
The oceanographers may not be able to measure every detail of the bottom relief in the entire ocean anytime soon, but they have a fairly good understanding of its statistical properties. They adopted the statistical representation of bottom roughness provided by actual echo-sounding systems. The NPS researchers realized that the key to the "perfect model" is to make topography as realistic as possible. This was tested out by old papers that didn't account for the bottom roughness in their models. Without accounting for small-scale topography, physics suggests that ocean rings should dissipate within a few weeks. I'm becoming skeptical of everything that assumes the bottom is smooth." "If this small-scale topography affects this vortex, it may affect currents, waves, and what not. Usually, topographic roughness is not even considered by theoreticians when looking at surface water activity. Scientists have been trying to figure out what makes large vortices stable and long-lived for about 50 years, but no one thought to look at the ocean floor's small-scale topography because it seemed too far away to impact these ocean rings. In the same manner, the small-scale texture of the seafloor slows down currents near the ocean bottom, which improves the stability and longevity of ocean rings near the surface. Radko and Gulliver call their finding the "sandpaper effect"-a moniker that draws association with the small abrasive particles of sandpaper that can grind down much larger objects. This is his first paper as a lead author." "It's like getting on the cover of Rolling Stone … You're a rockstar," Radko jokes. The research was significant enough to make the front cover of the journal (Volume 49, Issue 5) with a computer image of the model created by Gulliver as the main visual. "We need to remove systematic biases that numerical models have, and some of those are linked to the way models handle small-scale bottom topography," Radko explains.Įddies can create their own weather and wave patterns, and they can impact acoustics, among other things. This new understanding of how the ocean floor impacts surface currents will improve complex, numerical models used by the Navy's meteorology and oceanography (METOC) community to provide critical information to operational commanders.
#Naval nuclear laboratory cracked
Ocean rings are critically important for transporting heat and nutrients throughout the ocean and can last anywhere from a few months to several years.Īs detailed in the latest edition of the journal Geophysical Research Letters, it appears Naval Postgraduate School (NPS) Department of Oceanography doctoral student Larry Gulliver and Professor Timour Radko have cracked the code on exactly what makes some ocean rings last up to a decade while others dissipate within a few months: seafloor topography. Our measurements provide means to precisely assess our understanding of the fundamental baryonic interactions with strange quarks, which can impact our understanding of more complicated systems involving hyperons, such as the interior of neutron stars or exotic hypernuclei.One phenomenon that has stumped researchers for years is how swirls of circular currents multiple kilometers wide, known as ocean rings or eddies, stay intact. Transport models, combining baryonic mean-field and coalescence ( jam) or utilizing dynamical cluster formation via baryonic interactions ( phqmd) for light nuclei and hypernuclei production, approximately describe the measured H Λ 3 and H Λ 4 yields.
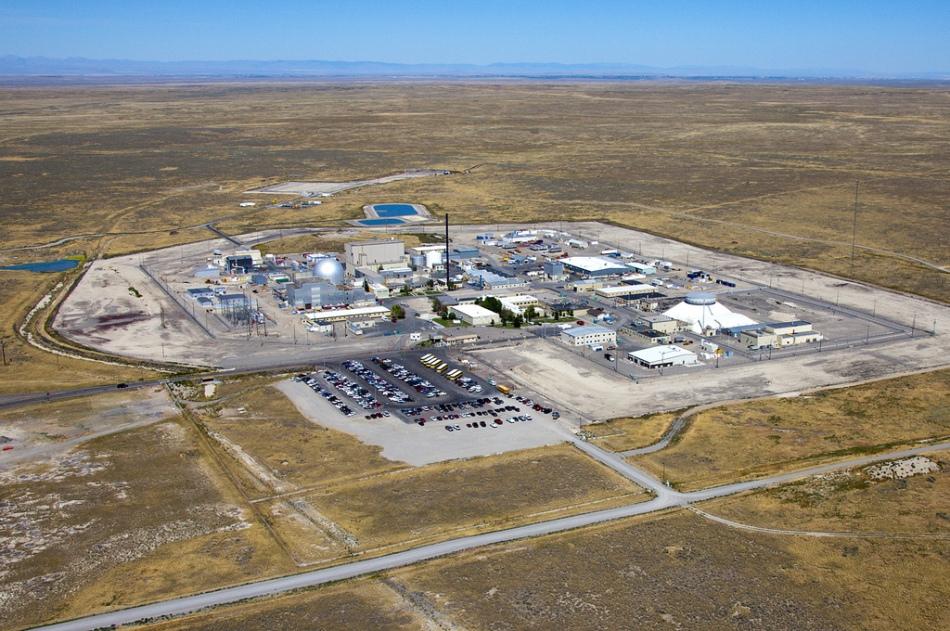
Thermal model calculations, using the canonical ensemble for strangeness, describes the H Λ 3 yield well, while underestimating the H Λ 4 yield. It is observed that the shape of the rapidity distribution of H Λ 4 is different for 0%–10% and 10%–50% centrality collisions. The p T-integrated yields of H Λ 3 and H Λ 4 are presented in different centrality and rapidity intervals. Their lifetimes are measured to be 221 ± 15 ( stat ) ± 19 ( syst ) ps for H Λ 3 and 218 ± 6 ( stat ) ± 13 ( syst ) ps for H Λ 4. H Λ 3 and H Λ 4, being the two simplest bound states composed of hyperons and nucleons, are cornerstones in the field of hypernuclear physics. We report precision measurements of hypernuclei H Λ 3 and H Λ 4 lifetimes obtained from Au + Au collisions at s N N = 3.0 GeV and 7.2 GeV collected by the STAR experiment at the Relativistic Heavy Ion Collider, and the first measurement of H Λ 3 and H Λ 4 midrapidity yields in Au + Au collisions at s N N = 3.0 GeV.
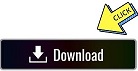